Omic.ly Weekly 66
March 10, 2025
Hey There!
Thanks for spending part of your week with Omic.ly!
This Week's Headlines
1) When a "rare variant" isn't actually rare
2) Genetic discrimination is still alive and well in the US
3) mRNA vaccines almost didn't happen
Here's what you missed in this week's Premium Edition:
HOT TAKE: Nautilus' failure to launch is now in its 4th season
Or if you already have a premium sub:
A lack of genetic database diversity highlights red herrings in disease diagnosis
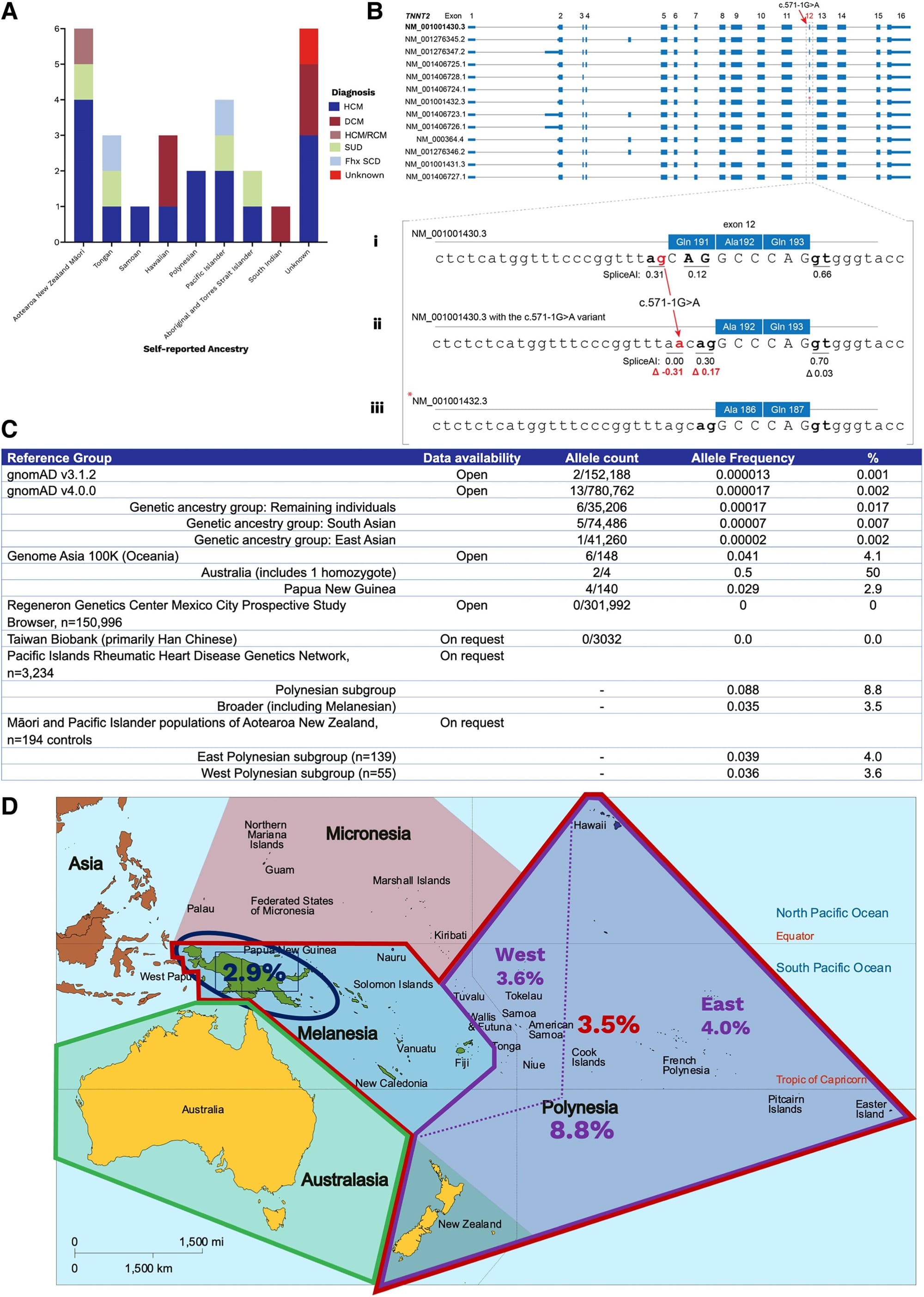
Each of our genomes has approximately 3 billion base pairs.
So, when it comes to diagnosing likely genetic diseases, that means that there's a lot of data to dig through.
That's because those 3 billion base pairs aren't the same in all of us.
We have variants that are unique to us as individuals and variants that are unique to each of our ancestries.
This means that if we compare my genome to your genome, we're going to see A LOT of differences in them.
Like, millions of differences!
And we know this because when we compare individual genomes to the standard reference genome that was sequenced as a part of the human genome project, we find, on average: 5 million single nucleotide variants (SNVs), 600,000 small insertions/deletions (indels), and 25,000 structural variants (SVs).
So you can imagine that trying to figure out which of those millions of variants could be the cause of disease in an individual is pretty challenging!
But we do have tools at our disposal, and when someone presents to a clinic with a likely genetic disease, there's a lot to consider.
Clinicians take into account things like phenotype or the visible features of a disease, or if there's a family history of disease, they try to determine the inheritance model (dominant/recessive).
This information is then used to decide what genetic mutations best fit with a disease.
For example, you wouldn't pick variants in genes that aren't directly related to a disease phenotype, nor would you pick genes whose impacts don't match the inheritance model.
But how do you even know that a variant could even be problematic?
Well, we look at whether variants are "damaging" and this is usually a measure of how much a variant potentially could change the structure of an expressed protein.
So things like deletions, insertions, and splice site mutations are typically the most damaging, but SNVs can also alter the function of proteins.
We also look at how well conserved sequences are across the tree of life, and mutations in highly conserved regions are usually prioritized over mutations in regions that aren't as well conserved.
The thinking here being that if a region of the genome hasn't seen much change over time, it's probably important!
But now that we have high throughput whole genome sequencing, and have sequenced many individuals across ancestries, we've seen that each ancestry has its own conserved variants.
This makes figuring out which variants could be causal of disease extremely challenging!
That's because the frequency of a particular variant could be low and highly damaging in one population, or that same exact variant could be common and totally benign in another!
This is why diversity in genetic databases is so important, because it allows us to refine our variant classifications based on ancestry and help us pick out which variants (out of millions) are most likely to be causing someone's disease.
And this isn't just some unsubstantiated concern in the field of clinical genetics.
We know for a fact that the case solve rate is much lower in non-white populations because we just don't have good enough information to make confident guesses about a variant's disease status in other populations that haven't been sequenced as much.
And if we don't have diverse databases, we also run the risk of calling some of those benign ancestral variants as disease causal!
A good example of this problem was recently published in the European Heart Journal where two unrelated patients of Oceanian ancestry presented to an Australian clinic with cardiac phenotypes and it was discovered that they both had the same mutation (c.571-1G>A) in Cardiac troponin T (TNNT2).
Mutations in TNNT2 have been shown to be associated with hypertrophic (HCM) and dilated (DCM) cardiomyopathy so the clinicians here decided to review whether this was a causal variant.
Their workup can be seen in the figure above where in A) they found 26 other unrelated individuals with this variant, many who were of Oceanian descent, B) displays all of the splice variants of TNNT2 - Bi, sequence of the most common splice variant; Bii, highlights the variant in question here; Biii, shows a rarer splice variant and that there's a cryptic splice site that becomes activated in the presence of the mutation in question (compare red numbers) - meaning, this variant is well tolerated and doesn't damage the expressed protein, C) is a table of allele frequencies from various databases showing MOST lack enough diversity to accurately classify this variant, D) is a map of Oceana highlighting the high prevalence of the variant in question by region.
This case report is an exquisite example of how variants can be red herrings in genetic diagnosis.
Variants are only considered rare when their population prevalence is <1% and the ACMG recommends classifying a variant as benign if its population prevalence exceeds 5%.
In the Oceanian population, this TNNT2 variant has a prevalence of 3-9%, meaning that as far as variants go, it's pretty common.
Indigenous island populations also have been shown to share about 3% of their DNA with neanderthals and these authors were able to find this variant in two archaic genomes (Vindija and Altai Neanderthal), indicating that it arose 130–145 thousand years ago which helps to explain why this variant is seen in Oceanian populations.
Studies like this underscore the importance of having highly diverse genetic databases and reference genomes that reflect all of the possible ancestral mixtures of variants that we might observe in the clinic.
###
Butters A, et al. 2025. A rare splice-site variant in TNNT2: the need for ancestral diversity in genomic reference data sets. European Heart Journal. DOI: 10.1093/eurheartj/ehaf001
Did you know that before 2008, insurers could discriminate against you based on your genetic risk for disease?
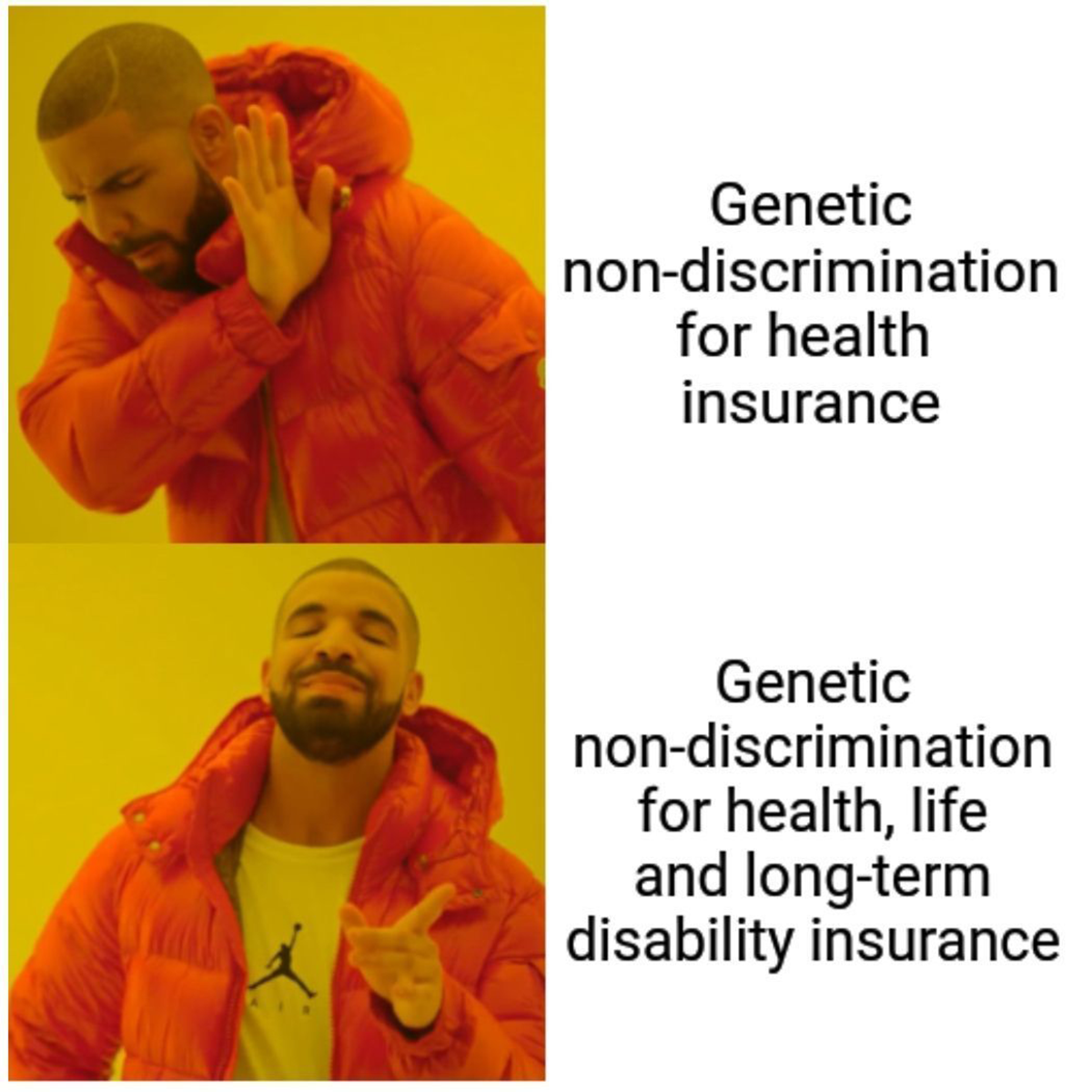
That ended when the Genetic Information Non-discrimination Act (GINA) was passed, but curiously, this law only applies to health insurance and employment.
These protections do not extend to life insurance, disability insurance, or long-term care insurance.
But we'll get to those in a minute.
You might be thinking that 2008 seems pretty recent for the passage of a major civil rights law, but that it coincides with when we 'finished' the human genome.
That kind of makes sense, right?
Unfortunately, genetic discrimination, like most discrimination, started a long time ago.
Beginning in the 1970's, some states forced African-Americans to get sickle cell tests with the intent to deny them health insurance or jobs if they tested positive.
These policies were blocked after 2 years but we had to wait 30 years for these sorts of protections to be enacted more broadly.
And that's because the use of widespread genetic testing opened everyone up to the same discrimination that had been perpetuated against African-Americans, so protective legislation was proposed and then put in place in 2008.
The politics here are a bit gut wrenching, but GINA has a pretty broad definition for what it considers a genetic test which is "an analysis of human DNA, RNA, chromosomes, proteins, or metabolites, that detects genotypes, mutations, or chromosomal changes."
GINA prevents insurers from denying anyone health insurance based on results from these genetic tests, and it prevents employers with more than 15 employees from denying anyone a job based on them too.
Wait, what? There's a 15 employee loophole?
Someone should fix that.
But what also needs fixing is how far GINA extends into the insurance industry because life, long-term care, and disability insurance companies can use genetic tests to deny insurance or raise rates.
These companies argue that they're selling risk based products and so it only makes sense that they're able to consider genetic risk when determining rates.
The problem is that genetics isn't deterministic, meaning, just because you have a mutation doesn't mean you're going to get a disease.
We know that genetics alone is very bad at predicting disease in healthy people, it's decent in determining a cause in someone showing symptoms but on average, 8% of people carry a pathogenic mutation, but only 7% of those will become symptomatic.
This is further complicated by the fact that gene-risk associations are highly dependent on the populations tested and so risk scores generally cannot be applied broadly or across ethnicities.
It is especially concerning if these bad or inappropriate tests are being used to unfairly deny coverage or increase rates.
It is estimated that COVID-19 vaccination has prevented over 20 million deaths worldwide. We can thank the dogged perseverance of a browbeaten scientist for that.
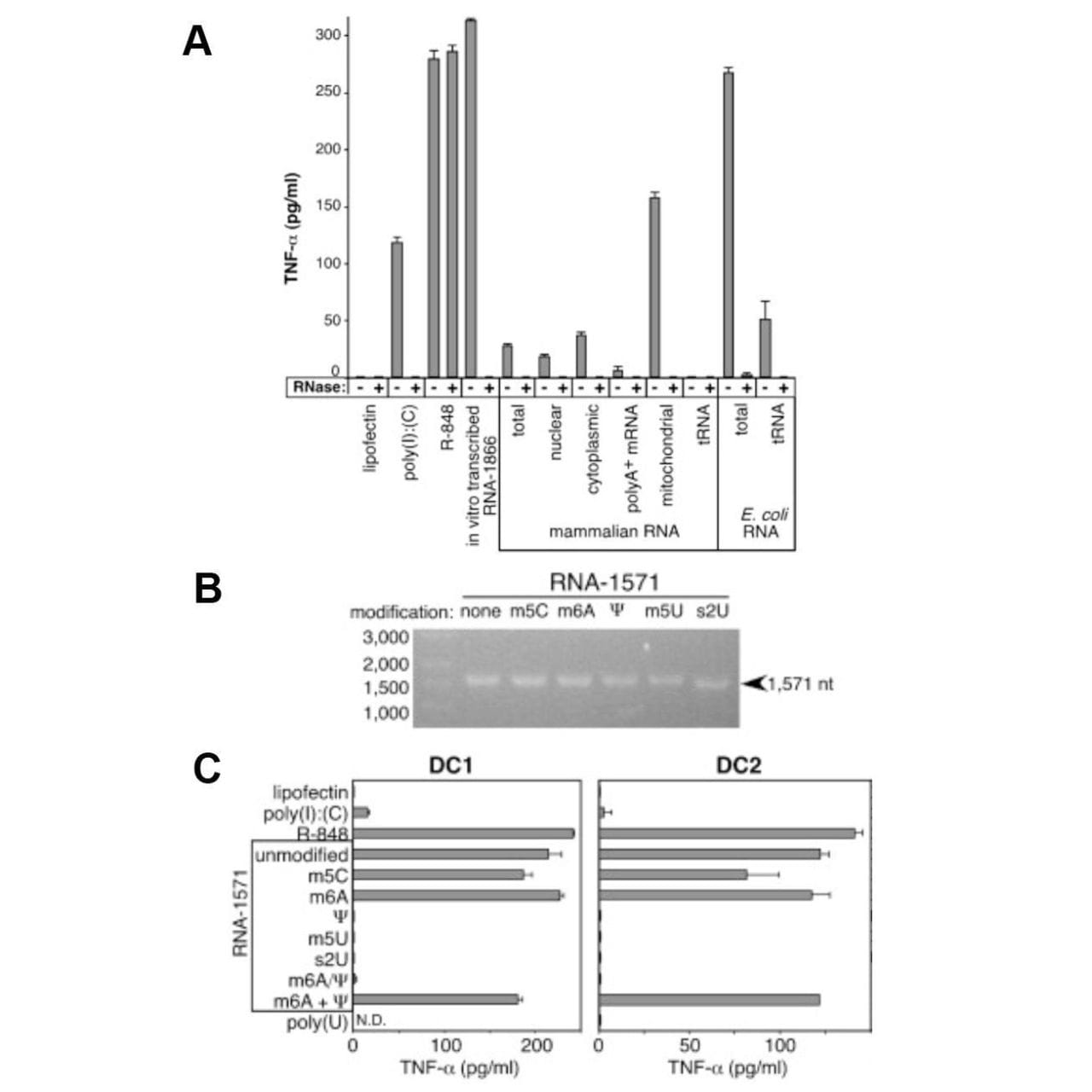
Katalin Karikó’s love of mRNA began during her postdoc at the Biological Research Centre of Hungary.
She had a feeling mRNA was destined for greatness, and moved to the US with her family in 1985 to continue her pursuit of turning it into a therapeutic.
However, as a foreign scientist in the US, she was often passed over for faculty positions and grants.
She persisted in spite of these setbacks.
Fortunately, a chance meeting with Drew Weissman led to one of the most important discoveries in modern medicine.
In 1997, Weissman had just completed his fellowship under the supervision of Anthony Fauci, and was in desperate need of some mRNA.
Karikó provided that mRNA but they noticed that it caused an inflammatory response in mice, which, unfortunately, would limit its usefulness as a therapeutic.
Undeterred, the pair decided to figure out why.
The figure above shows what they discovered.
In A, Dendritic Cells (produce TNF-α, an immune cytokine) were treated with 3 controls (poly I:C - synthetic RNA, R-848 - a drug, and in vitro transcribed RNA) which are known to stimulate the immune system along with isolated mammalian RNAs, and bacterial RNAs.
Only mitochondrial RNA and bacterial RNA induced a strong response (in addition to the controls), while tRNA had no effect!
So, what accounts for this difference?
Karikó and crew thought that since 25% of tRNA bases are modified, maybe that’s what prevented most of the mammalian RNAs from sounding the alarm!
In B, mRNA was transcribed using modified nucleotides: 5-methylcytidine (m5C), N6-methyladenosine (m6A), pseudouridine (Ψ), 5-methyluridine (m5U), or 2-thiouridine (s2U).
And in C, those mRNAs were introduced into dendritic cells.
The modified uridine containing mRNAs failed to cause an immune response.
Success!
Karikó later showed that Ψ containing mRNA produces the most protein and that those transcripts last for up to a month.
However, neither the institution where she was employed nor the US government recognized the tremendous potential of her work.
And so, she left the 'Mean Girls' culture of US academia and joined a little German company called BioNTech.
She continued her collaboration with Weissman and, with BioNTech, developed the first experimental vaccine using modified mRNAs against Zika in 2017.
BioNTech went on to produce the first mRNA vaccine for SARS-CoV-2 with doses being delivered worldwide in less than 8 months.
The recent value of this achievement can be measured in tens of millions of lives, but its future has the potential to save hundreds of millions more.
Karikó and Weissman were awarded the Nobel Prize for this work in 2023.
###
Karikó K et al. 2005. Suppression of RNA Recognition by Toll-like Receptors... . Immunity. DOI: 10.1016/j.immuni.2005.06.008
Were you forwarded this newsletter?
LOVE IT.
If you liked what you read, consider signing up for your own subscription here: