Omic.ly Weekly 60
January 27, 2025
Hey There!
Thanks for spending part of your week with Omic.ly!
This Week's Headlines
1) A foundation model for transcription in all known cell types
2) Multi-omics and drug therapeutic development: A match made in heaven
3) The discovery of sex chromosomes was just as controversial as everything else in genetics
Here's what you missed in this week's Premium Edition:
HOT TAKE: Will Truveta out helix Helix?
Or if you already have a premium sub:
A new foundation model has been trained that predicts transcriptional activity in all known cell types!
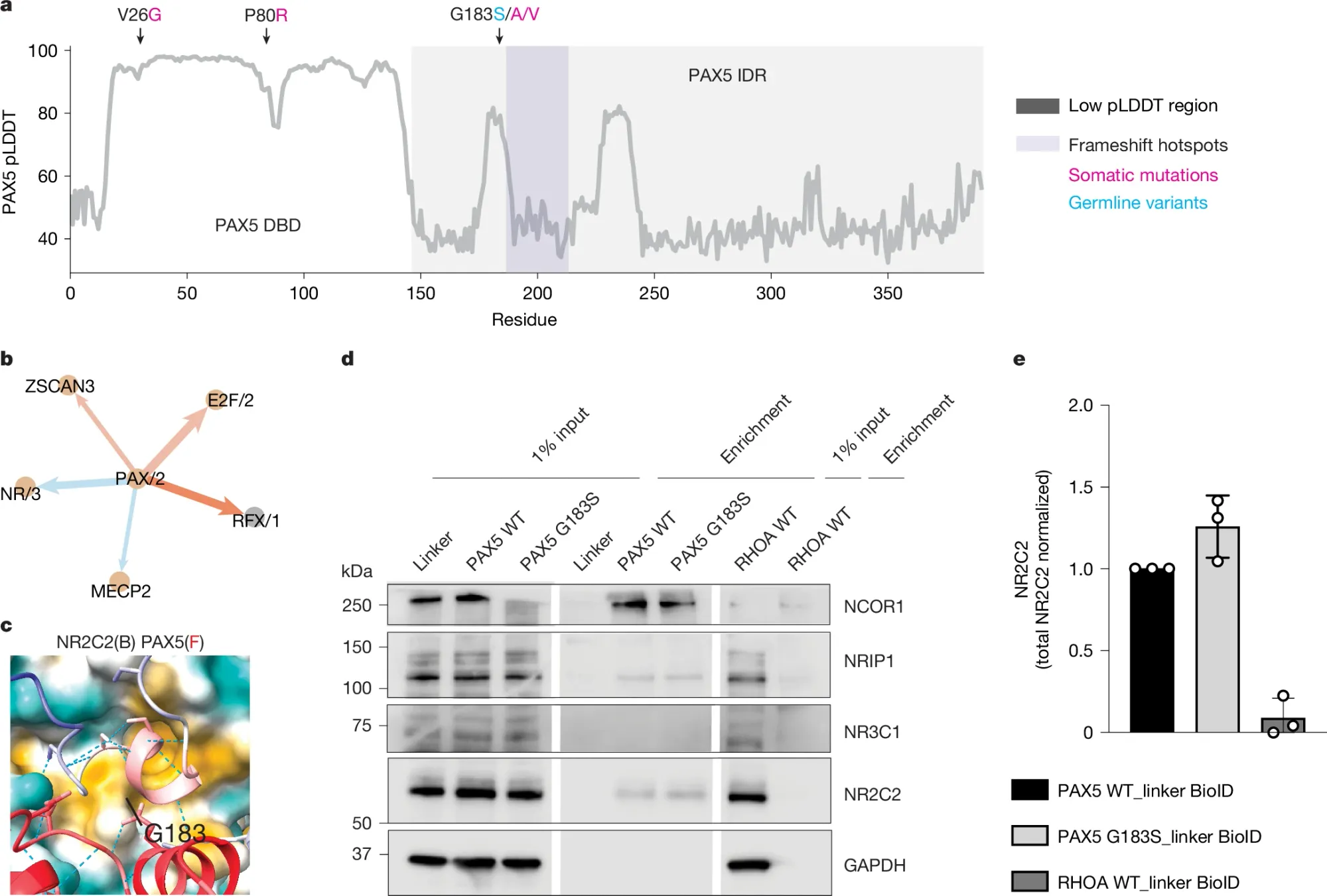
“But, does it make those predictions accurately?”
Shhhh, we’ll get to that, don’t spoil the fun!
First we need to define “transcriptional activity” which in itself is a crazy complicated topic.
But for brevity, transcription includes all of the things that operate together in the process of converting the genetic code into an RNA message.
This includes things like the binding of transcription factors to each other and to sequence motifs in DNA, the opening of chromatin to allow those things to bind, and the recruitment of an RNA polymerase to do the transcribing.
And it’s the transcription of DNA into an RNA message and its subsequent translation to protein that drives most of the activity in our cells.
Transcription is also one of the major factors that helps to determine the function of a cell and its role within a tissue.
To date, we’ve identified over 200 different cell types in our body and all of these interact to create the tissues and organs that make us who we are!
But how our DNA code can be manipulated to create all of these different cell types is still largely unknown.
We’ve made some progress figuring this out though, and new single-cell techniques combined with high throughput sequencing are providing us with more data than we’ve ever had on this topic.
And thanks to research consortiums like the Genotype-Tissue Expression project (GTEx), this data is all publicly available for researchers to dig into to develop new models of gene expression!
A number of gene expression models have been developed for specific cell types, but the researchers behind today’s paper decided to go the foundation model route (which attempt to make generalized predictions based on their training data).
What they produced was a model based on “chromatin accessibility data across 213 human fetal and adult cell types [that] accurately predicts gene expression in both seen and unseen cell types.”
They named it General Expression Transformer (or GET for short) and showed that it does a reasonably good job of predicting cell type specific gene expression (r2=.88 vs mean expression level - .6, transcription start site accessibility - .22, and gene activity - .26),
But making gene expression predictions in unknown cell types isn’t GET's only trick!
The researchers showed that it can also predict regulatory activity, identify cis-regulatory elements (the DNA sequence kind), and predict interactions between transcription factors.
That’s a lot of information to get out of a model trained ONLY on chromatin accessibility data!
But, what’s the point of a foundation model if you can’t show it actually does something useful?
So, they put GET to work trying to figure out how a variant (G183S) in a transcription factor, PAX5, facilitates B cell precursor acute lymphoblastic leukaemia (B-ALL).
In a) they show that the G183S variant is in the intrinsically disordered region (IDR) of PAX5 b) GET predicted B-Cell sequence motif interactions includes PAX5 and NR/3, a common nuclear receptor domain c) AlphaFold predicted structure of the PAX5 IDR bound to an NR domain d) Binding experiment showing that PAX5 sticks (middle panel) to NCOR1, NRIP1, and NR2C2 e) The G183S PAX5 variant appears to make PAX5 bind better to NR2C2 (maybe…)
Despite the fact that all of these interactions were previously known, GET could be useful in prioritizing the follow-up of druggable pathways in diseased cells.
We can debate the utility of GET in its current iteration (I'm in the "meh" camp) but the authors suggest that training on more than just chromatin accessibility data (like perturb-Seq) will help to get GET better at predicting the transcriptional regulatory frameworks hiding within our DNA.
###
Fu X, et al. 2025. A foundation model of transcription across human cell types. Nature. DOI: 10.1038/s41586-024-08391-z
The drug therapeutic landscape expands almost daily. Pairing them with multi-omics is a no brainer!
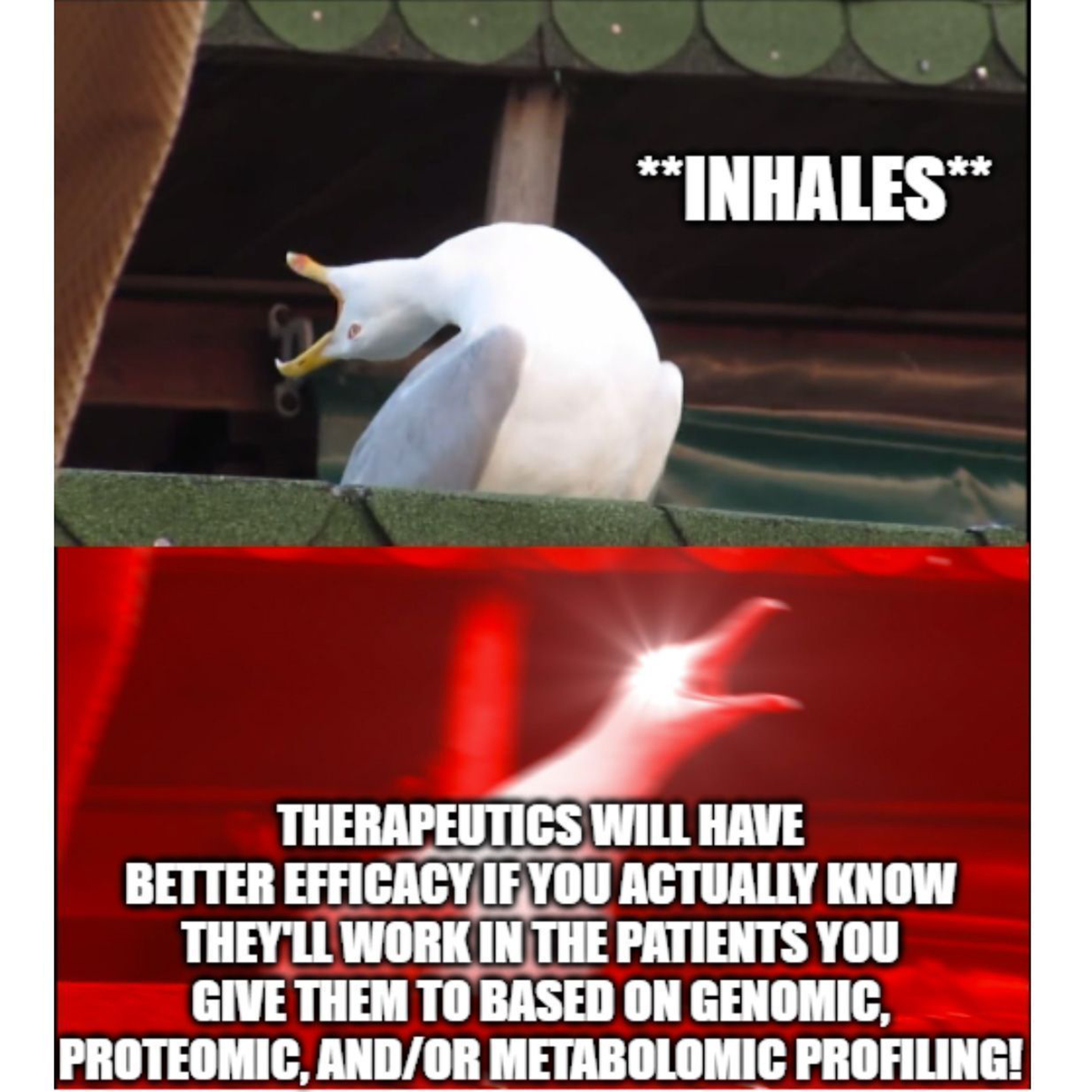
That isn't rocket science.
But what IS rocket science is developing the tests that make sure those therapies work, and continue to work, when administered to patients!
We do have some pretty good experience with this sort of thing already though.
Technologies such as pharmacogenetic testing have emerged to help predict how patients will respond to certain classes of drugs.
This is done by looking at genetic markers that indicate how quickly someone might metabolize, absorb, or eliminate a drug!
But we also have experience developing ‘companion diagnostics.’
These are diagnostic tests that are used to place patients on a specific therapy.
The first of these was used in 1998!
HercepTest was introduced to identify patients who would respond best to Herceptin, an early antibody treatment for breast cancer.
This test was necessary because Herceptin only worked in patients if their tumors overexpressed the HER2 receptor!
And because of this integration with a biomarker test, Herceptin is often referred to as the poster child for precision medicine!
But we’ve come a long way since Herceptin, and there are some really cool new precision therapeutics on the horizon:
PROteolysis TArgeting Chimeras (PROTACs) - Small molecule drugs that have one end that binds to a target protein and another that binds to E3 Ubiquitin Ligase (a protein that marks other proteins for destruction!)
Antibody Drug Conjugates (ADCs) - These are antibodies that are physically bound to drugs to make their delivery more targeted. The biggest successes here have been in targeting chemotherapy drugs to tumors!
Translation Activating RNAs (taRNAs) - RNA molecules designed to bind to a target RNA to supercharge its translation. This is done by adding a sequence called an Internal Ribosome Entry Site (IRES). These boost ribosome binding on the target RNA and increases production of the target protein.
mRNA Vaccines - We're all familiar with these, but what you might not know is that they can also be quickly programmed to create personalized cancer treatments.
Chimeric Antigen Receptor - T cells and Macrophages (CAR-T/M) - Are immune cells that have been programmed or personalized to seek out and destroy tumor cells.
These are all very exciting, but their development requires a lot of testing to tailor each treatment to an individual.
But what excites me the most in this space is getting the opportunity to move beyond the single biomarker tests of old!
Because seeing the full picture of a patient's response to a therapy through expanded proteomic and metabolomic screening could:
1) Show us how well a drug is working
2) Signal when someone will relapse
3) Mitigate side effects before they're felt
4) Indicate when to change therapies
This is the version of precision medicine that we were promised and I’m hopeful we see these applied more broadly in the clinic soon!
Nettie Stevens, a former school teacher turned geneticist, discovered sex chromosomes in 1905. Here's her story:
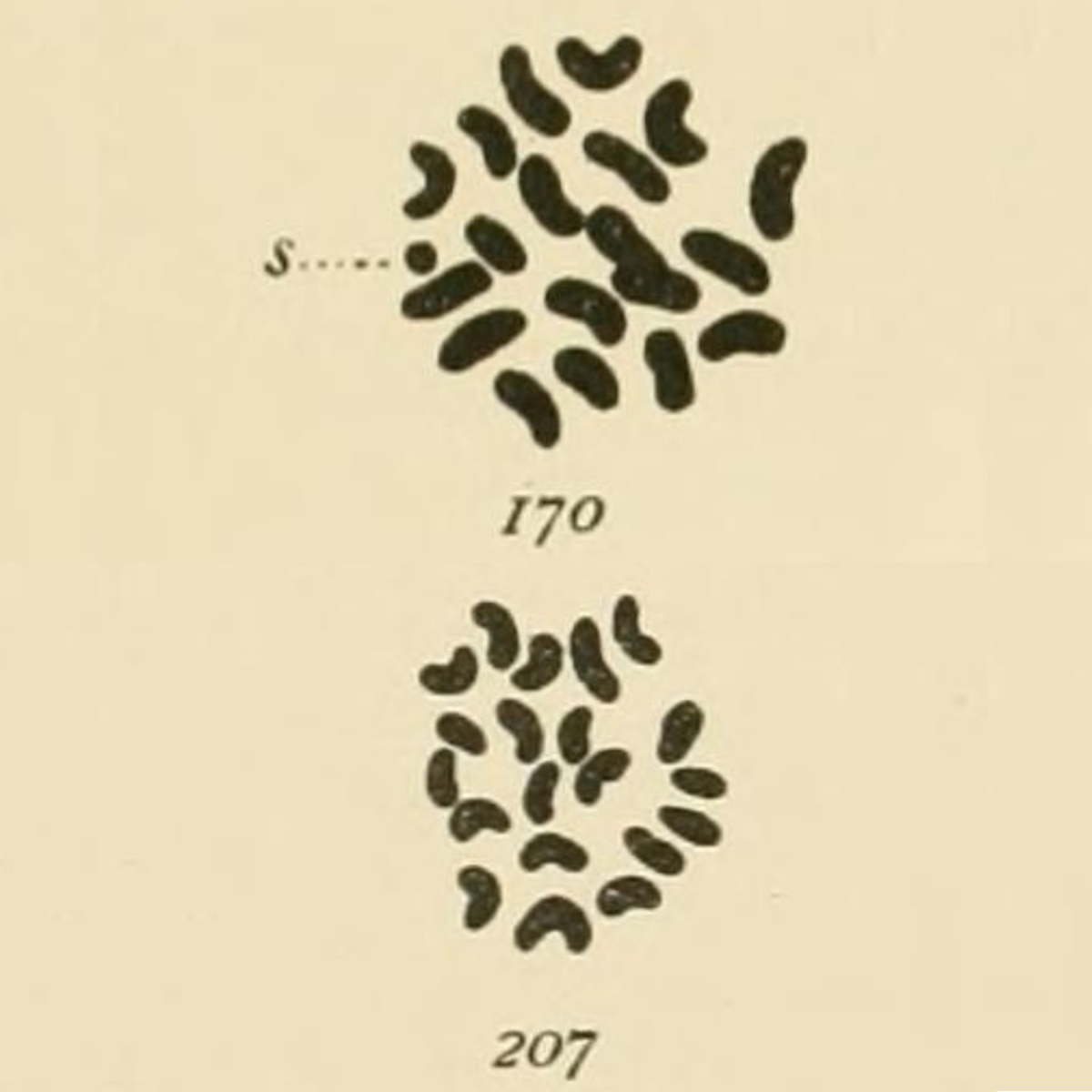
The late 1800s were a turning point in American history for women.
The end of the Civil War marked the start of the women's rights movement and granted them significantly more control over their own lives.
That being said, women were still expected to be teachers or home makers but they won more personal freedom including better access to education.
Stevens took advantage of these expanded liberties, and started her educational pursuits at age 10 studying to become a school teacher.
In 1883, she began a decade-long career in education, filling roles both as a teacher and as a librarian.
However, this wasn't her life's dream, and in 1896, at the age of 34, she had saved enough money to enroll at Stanford University earning both her bachelor's and master's degrees in 1900.
It was during her summer studies that she took a keen interest in cytology while working at the Stanford Marine Lab.
Here she spent her time glued to a microscope and published her first paper on the life-cycle of ciliates in 1901.
Stevens then left Stanford to continue her scientific studies at Bryn Mawr beginning her dissertation work under the guidance of Thomas Hunt Morgan.
You might be familiar with him.
Morgan received the Nobel Prize in 1933 for his work elucidating the role of chromosomes in heredity.
Interestingly, Morgan was initially skeptical of heredity, particularly as it related to sex determination.
At the time, there were two competing hypotheses:
One being that sex was determined by environmental factors, like temperature, and another that sex was inherited as a trait.
In spite of the lingering questions on this topic, Stevens successfully completed her dissertation in 1903, and received an award from the Carnegie Institution to study how sex is determined.
This work is the subject of the figure below wherein Stevens meticulously detailed the cellular structures of the reproductive organs of multiple insects.
The most striking of these being her drawings in 1905 of the chromosomes found in mealworms (Tenebrio molitor) where she observed:
‘In both somatic and germ cells of the two sexes there is a difference not in the number of chromatin elements, but in the size of one, which is very small in the male (170-s) and of the same size as the other 19 in the female (207).’
Even though she was the first to make this discovery, Thomas Hunt Morgan and Edmund Beecher Wilson are often incorrectly given the credit.
Tragically, breast cancer cut her scientific career short at the age of 50.
But Nettie Stevens' contributions to our understanding of sex determination, along with her exquisite drawings, were the definition of groundbreaking in the field of genetics.
###
Stevens NM. 1905. Studies in Spermatogenesis with Especial Reference to the Accessory Chromosome. Carnegie Institution.
Were you forwarded this newsletter?
LOVE IT.
If you liked what you read, consider signing up for your own subscription here: