Omic.ly Weekly 58
January 13, 2025
Hey There!
Thanks for spending part of your week with Omic.ly!
This Week's Headlines
1) Keeping things separated: How our brains prevent erasing old memories
3) Epigenetics and transcription are so intertwined it's hard to tell them apart
4) The first human Mendelian disease to be described
Here's what you missed in this week's Premium Edition:
HOT TAKE: Ultima Genomics and Olink nab a population scale project
Or if you already have a premium sub:
Why new memories don't overwrite old ones (in mice, and hopefully humans, too)
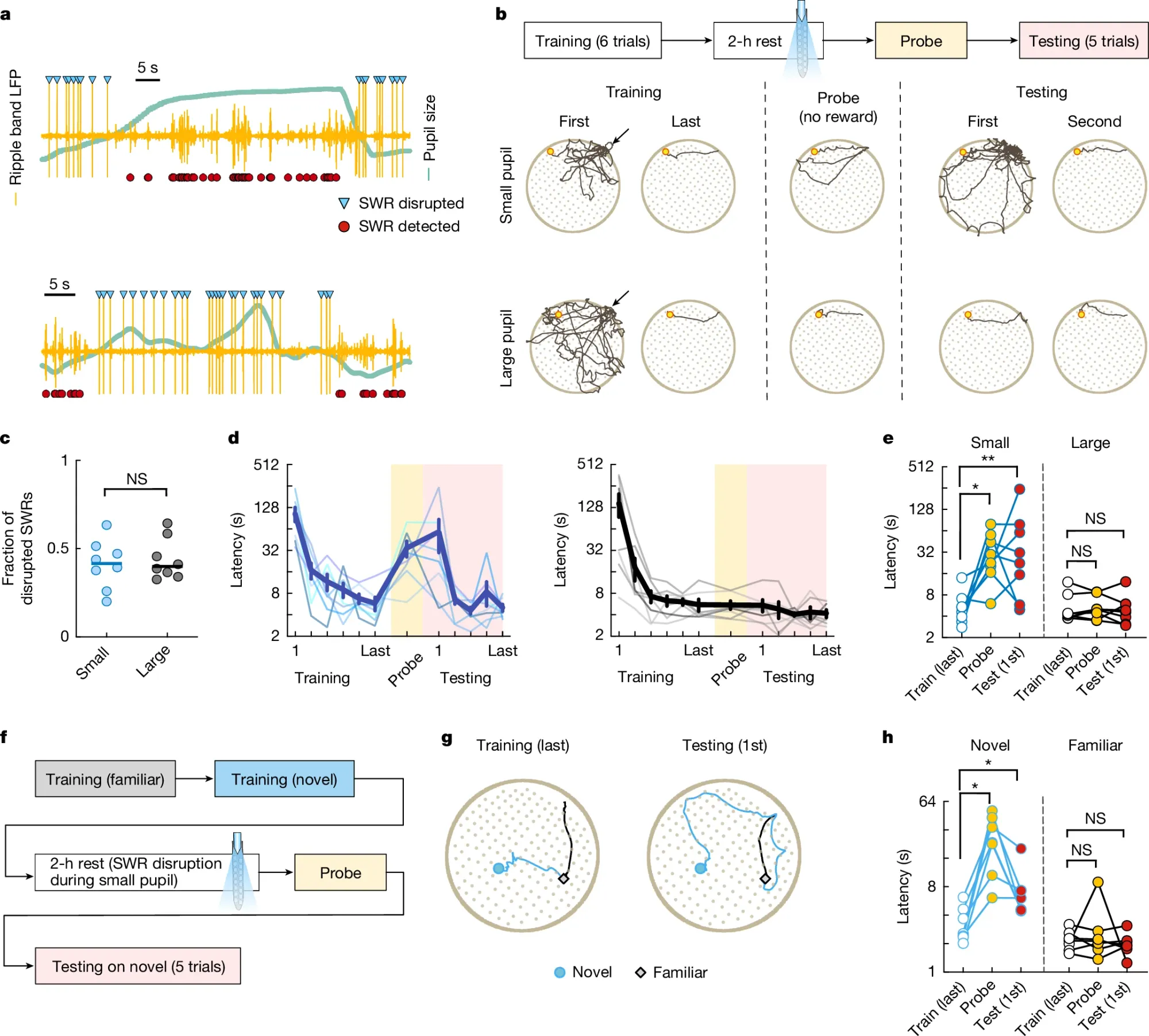
Have you ever wondered why you're able to remember anything at all?
It's because we have memory!
Memory is a highly conserved evolutionary feat in animals.
And it's not restricted to just mammals - birds, fish, and even lizards can form and retain memories.
Memory is hugely beneficial to complex organisms because it allows them to recall positive and negative situations that they encounter.
And even if those memories are unpleasant, it can be good to retain them so that you avoid that unpleasantness in the future!
But one thing we've struggled to understand is how memories don't get overwritten.
It's been hypothesized that our brains segment the learning of new memories and the retention and replay of old memories so that these activities don't interfere with one another.
Because when they do collide, animals (and artificial neural network algorithms) experience a phenomenon known as 'catastrophic forgetting.'
That sounds as bad as you think it sounds.
So, understanding how our biological neurons make new memories and retain old ones is important.
Because we can apply what we learned in biological brains to prevent our artificial ones from catastrophically forgetting!
Quite a bit of research has been done on memory generation in biological brains and we know that most memory formation happens while we're sleeping.
Sleep allows us to replay the events of our day in our mind, and store the most interesting bits.
This seems to happen during non rapid eye movement (NREM) sleep and the replaying of memories (new and old) occurs in short wave ripple events (SWR).
However, was not known how these events were segmented, what memories were played when, or how our brain is able to retain old memories without overwriting them with new ones!
And while rapid eye movement (REM) and NREM sleep are important for cleaning out the garbage in our brain and storing memories, they're not the only important eye hallmarks of brain activity.
Researchers have also noticed that animal pupils dilate during NREM sleep and the researchers behind today's paper hypothesized that pupil dilation during NREM might be related to memory storage.
To test this, they engineered mice whose brain waves could be disrupted using a technique called optogenetics (use blue light to turn neuronal genes on and off).
In the figure above, you can see the results of their experiment a) schematic showing how they detected and disrupted (blue triangles) SWRs (red dots) that occurred when pupils (green line) were small (top graph) versus when they were big (bottom graph). b,d,e) shows the effect of SWR disruption on learning the location of a food reward in a maze - disruption of SWRs during small pupil events made the mice forget where the food was! c) this effect was not because there were more small pupil SWRs than large pupil SWRs and f, g, and h) show a second experiment where disruption of small pupil SWRs only affects recall of novel food locations and not memory of ones the mice had already learned previously.
The results of this study are important because they confirm the hypothesis that the brain is able to make and retain memories by segmenting the learning of new memories and the remembering of old memories in two different brain activity phases.
In mice, new memories appear to be solidified during small pupil SWRs.
And this is important because learning how our brains are able to perform these memory feats without mixing everything up could help inform how we develop the next generation of artificial intelligence algorithms.
###
Chang H, et al. 2024. Sleep microstructure organizes memory replay. Nature. DOI: 10.1038/s41586-024-08340-w
A molecular mystery: Is it epigenetics or is it transcription? Yes.
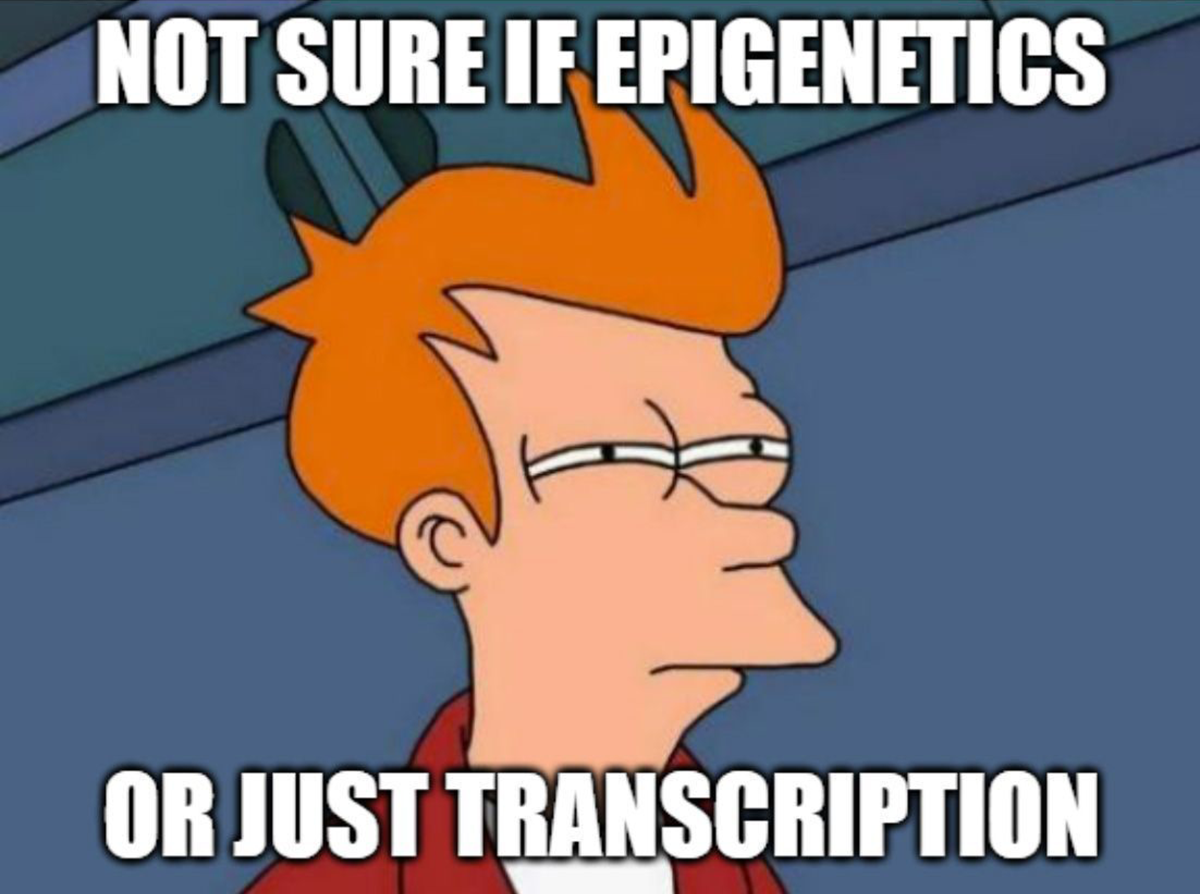
To say that epigenetics is both complex and confusing is an understatement.
There is a long-standing debate over what is considered ‘epigenetic.’
The technical definition of epigenetics is that it’s the study of alterations to DNA that do not involve changing the coding sequence.
These include cytosine methylation and histone modifications.
Histones are the little hockey pucks that coil up DNA into the chromatin that makes up our chromosomes.
And, epigenetic modifications work together to regulate access to DNA by physically closing off and packing it up into heterochromatin or relaxing and opening it to form euchromatin.
These changes ultimately help regulate which genes are expressed.
BUT for these to be considered ‘epigenetic,’ they must be passed onto daughter cells after cell division.
If the changes aren’t preserved, they’re considered ‘transient’ or temporary.
However, whether they’re transient or permanent, they regulate the transcription of DNA into RNA and this controls what proteins are made.
But things get weirder.
Because transcription itself can regulate how histones and DNA are modified.
The act of transcription is often enough to prevent the closing of DNA and keep it active!
And much of the machinery that is involved in maintaining chromatin structure and epigenetic modifications also play a significant role in recruiting all of the factors that result in the production of RNA!
But the opposite is also true.
If a region isn’t actively being transcribed, it’s much more likely to be closed off!
So, what is epigenetics, and what is transcription?
It can get very blurry.
But if we stick to the classic definition, epigenetics is just the stuff that sticks around through cell division.
So how does that actually work?
We’re still trying to figure it out, but it appears to be a combination of factors.
During cell division, a cell splits in half to create two new cells.
To do this, DNA has to be copied or replicated.
And in that process a new DNA strand is made.
As that copying occurs, factors recognize which regions are methylated on the parent strand and methylate the new strand.
The preservation of histone modifications is much more complicated and they can be similarly maintained during replication or they can be re-established later.
1. How much later?
2. And does that mean they’re still epigenetic?
Those are both good questions!
Because another way we know these modifications are preserved is by the continued association and/or eventual recruitment of…
**Drum Roll**
TRANSCRIPTION FACTORS!
This is called 'gene bookmarking' and it helps make sure that cells express the right genes after cell division.
But that brings us back to the original question about what is epigenetic and what is ‘just’ transcription.
The answer is that it’s context dependent.
But also, a lot of the time, the answer is also ‘Yes.’
Mendel first described his laws of genetic inheritance in 1865. They were promptly ignored for 35 years.
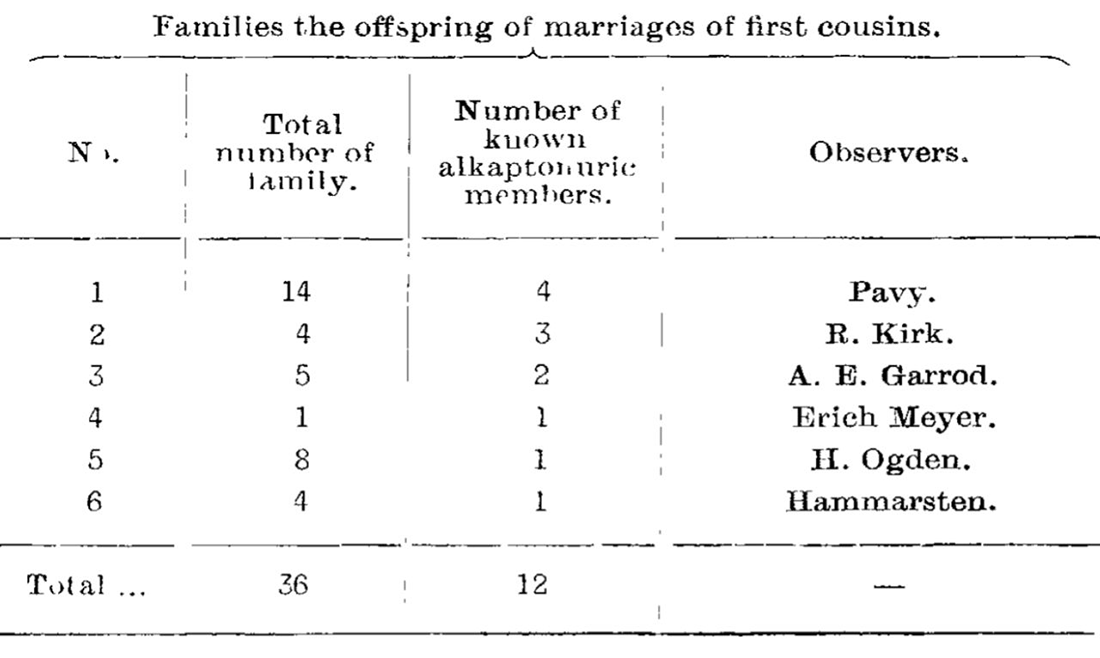
Because, let's be honest, who gives a husk about peas?!?!
It probably also didn't help that his paper was titled, 'Versuche über Pflanzenhybriden.'
Which in English translates to, 'Experiments in plant hybridization.'
While this sounds exhilarating, it belies the foundational concepts described in its pages and Mendel's work breeding peas wasn't revisited until 1900 when his results were independently rediscovered and confirmed by E. Tschermak (peas), W. Spillman (wheat) and C. Correns (peas).
H. de Vries (flowers) also independently characterized plant genetics in 1900 but had to be told that Mendel scooped him 35 years earlier.
Oof.
So, what was it that Mendel discovered while studying peas?
He observed the physical traits of peas and how these traits were passed to their offspring after breeding.
Mendel established 3 genetic principles from these observations:
Segregation - Traits come in two forms but only one from each parent is passed to offspring.
Independent Assortment - The segregation of the forms of each trait occurs independently of any other trait.
Dominance - Dominant forms of each trait mask the recessive forms and they occur in a 3:1 ratio.
Mendel initially described these as laws, but we know now that there are numerous exceptions, so in genetics we often refer to Mendelian and non-Mendelian inheritance.
But in the early 1900's, the race was on to see if Mendel's phenomenon wasn't just some weird plant thing.
One of the biggest proponents of Mendel at the time was William Bateson.
Bateson is a forgotten figure nowadays, but he popularized the works of Mendel and also did some of the earliest work on genetic linkage.
He also became fast friends with a clinician scientist, Archibald Garrod.
Garrod was most interested in the biology of the diseases in his patients and had a particular fondness for chemistry.
This could be why he was so enamored with the color of his patients’ urine.
This curiosity paid off in 1899 when he first noticed a chemical aberration in the urine of patients with Alkaptonuria.
This is an ancient disease with symptoms being described as far back as 1500 BC, but one of its tell-tale signs is darkly pigmented pee.
Garrod noted to his friend Bateson that this disease was found most often as the result of marriages between first cousins, and at Bateson’s urging, Garrod documented the incidence of Alkaptonuria in these families.
The figure above is the first evidence of a recessive Mendelian disease in Humans.
In the table you can see that in these families the dominant form of the trait is observed in 36 offspring, and the Alkaptonuria form in 12.
This perfectly aligns with the 3:1 ratio Mendel first observed in his peas!
###
Garrod AE. 1902. The incidence of alkaptonuria: a study in chemical individuality. The Lancet. DOI: 10.1016/S0140-6736(01)41972-6
Were you forwarded this newsletter?
LOVE IT.
If you liked what you read, consider signing up for your own subscription here: