Omic.ly Weekly 47
October 28, 2024
Hey There!
Thanks for spending part of your week with Omic.ly!
This Week's Headlines
1) Cutting calories extends life, but not for the reasons you might think
2) Single-cell and Spatial Transcriptomics: All the cool kids are doing it
3) The most beautiful experiment in biology
Here's what you missed in this week's Premium Edition:
HOT TAKE: At-home testing companies were put on notice with FDA's most recent warning letter to Molecular Testing Labs
Or if you already have a premium subscription:
Would you cut 40% of your caloric intake if it meant you'd live 40% longer?
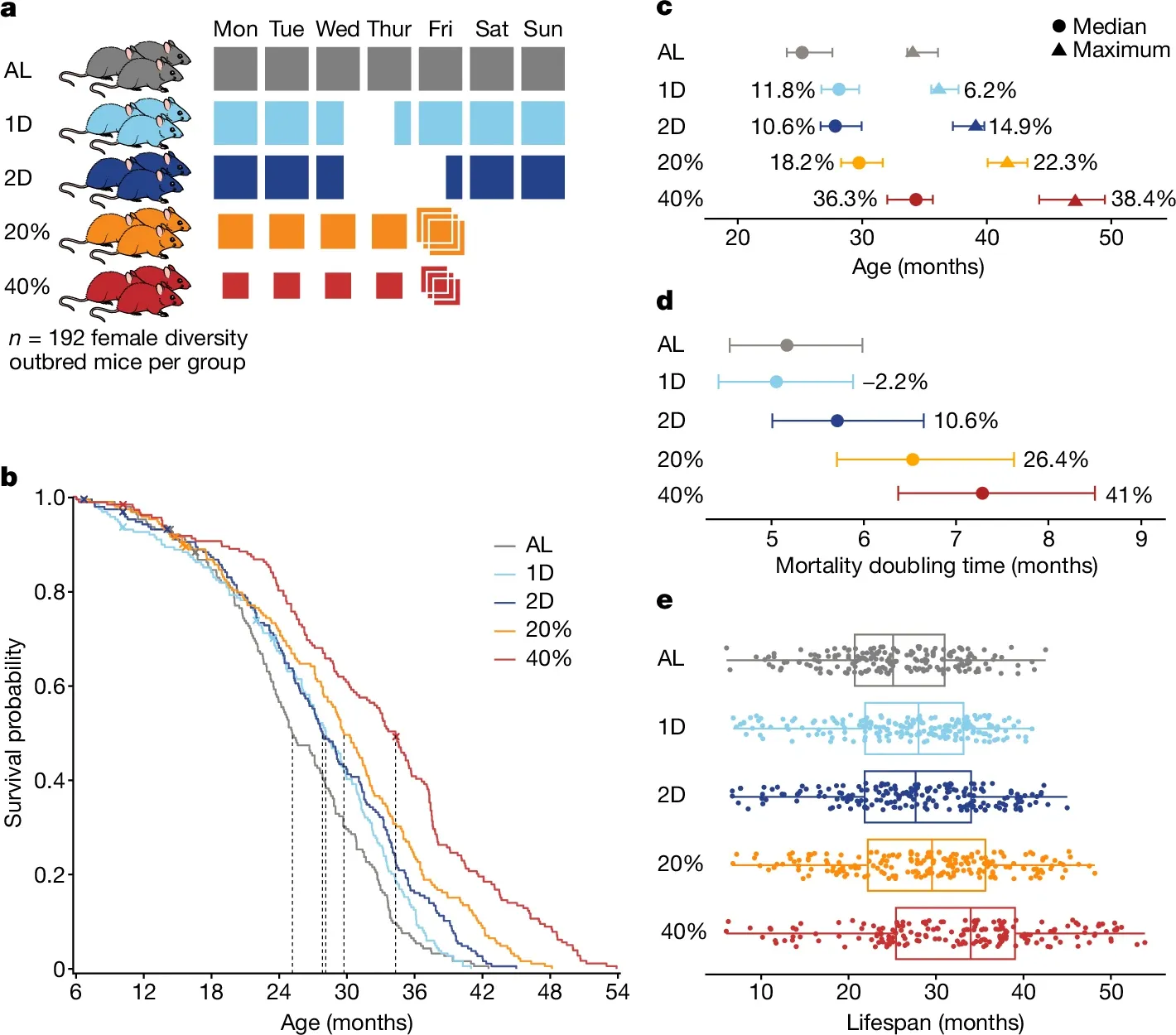
Well, if your answer to that question is yes, there's good news...
If you're a mouse, that is!
For the rest of us, there's still a bit of work to do.
With respect to people living in the United States, the average lifespan for males is 75 years and for females it's approximately 80 years.
Prior to 1800 and the introduction of modern medicine, human life expectancy was about half that.
By historical standards, we're doing pretty well!
But, humans have been trying to figure out ways to live even longer be it through leading healthier lifestyles or 'hacking' their own biology.
A lot of contemporary advice is centered on improving personal habits around diet and exercise.
So it's encouraging that recent studies in animals have shown that calorie restriction appears to increase lifespan.
The assumption here has always been that calorie restricted diets result in weight loss that promotes healthier metabolic function thus leading to longer lives.
At least that's what conventional wisdom has hypothesized.
But now new research in over 1,000 female mice has shown that this simplistic assumption is probably far more complex than we had initially thought!
In the figure above you can see a) mice were split into 5 groups: AL - Ad libitum (could eat as much as they wanted), 1D - fasted 1 day a week, 2D fasted 2 days a week, 20% - were given 20% less food, 40% - were given 40% less food b) Kaplan-Meier curves of survival by diet, dashed lines are median survival c) The median and maximum life expectancy of all groups undergoing dietary restrictions was increased d) Mortality doubling time increases (longer doubling times are better when it comes to death) for all diet restricted groups except for the 1 day fasters and e) Box plots showing the lifespans of all of the mice in each group
Ok, so case closed, calorie restriction can increase lifespan 30-40% (in mice)!
But these researchers weren't just interested in replicating the results of previous research by doing a bigger study, they were also interested in understanding WHY this happened.
Surprisingly, what they found was that metabolic traits were very poor predictors of lifespan and that mice who stayed chonkier while on the calorie restricted diets appeared to fare the best.
They also found that immune and haematologic (blood) traits were far better predictors of whether a mouse would live longer on a calorie restricted diet and that a mouse's genetic background accounted for a greater proportion of the variance in lifespan than the diet that they were placed on!
So, what does this all mean?
Calorie restriction in mice can generally make them live longer, but for mice looking to go the distance, it's all in their genes!
And, what does this mean for you and me?
It's probably the same, and with the holidays just around the corner, maybe now is a good time to think about cutting back (and taking stock of the age of your eldest relatives!)
###
Francesco AD, et al. 2024. Dietary restriction impacts health and lifespan of genetically diverse mice. Nature. DOI: 10.1038/s41586-024-08026-3
Single cell and spatial transcriptomics: What you need to know!
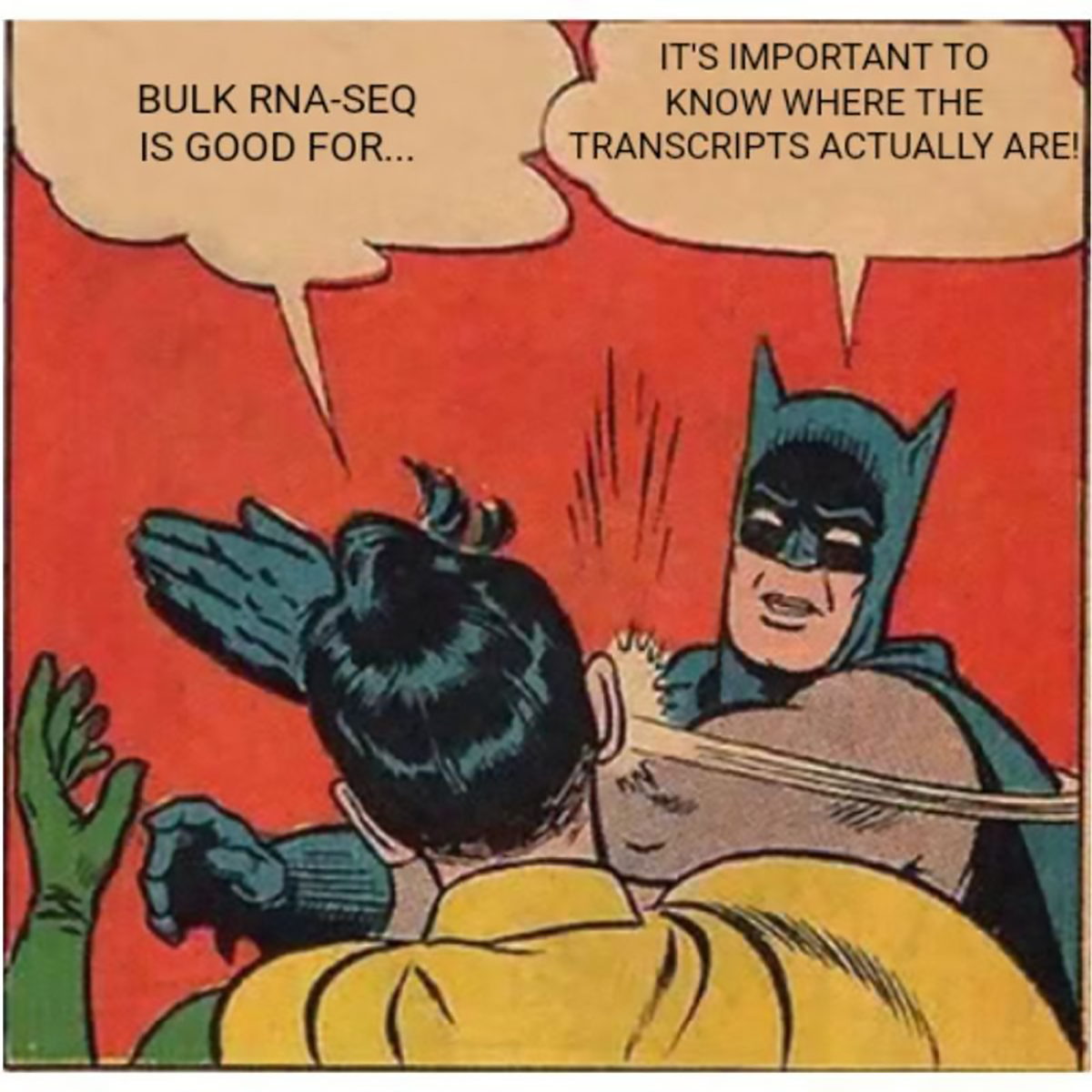
They're freaking cool!
The transcriptome is the study of all of the ribonucleic acid (RNA) produced within a cell.
It differs from the genome in a couple of important ways:
1) The transcriptome is made up of RNA messages, and the genome is made up of DNA
2) RNA is the template for making proteins, the genome is the template for making RNA
3) Each cell has the same genome but a different transcriptome
4) Each tissue (many cells working together) has a variety of transcriptomes that enable a function ie organ tissue
But for a very long time, our evaluation of transcriptomes were limited to surveying large populations of cells from ground up tissues or looking at whole blood.
We even have two consortiums dedicated to better understanding transcriptomes. One is the ENCODE Project or the ENCyclopedia Of Dna Elements and the other is the Genotype-Tissue Expression (GTEx) Project. Both projects have generated datasets that try to figure out how a single genome ends up creating the cellular diversity we see in all of our tissues by looking at regulatory elements in the DNA, protein associations with chromatin, epigenetic signatures, expression levels of RNA, and quantifying protein levels.
But, the initial datasets for ENCODE were based on sequencing homogenized tissues. This is a bit like trying to hang a picture with a sledgehammer when you don't have a tack hammer handy.
Thankfully, we can do more refined analyses now using single cell and spatial transcriptomics!
Single cell: This method was popularized academically as Drop-seq. It uses bead linked oil emulsions to capture transcripts from single cells for sequencing. Basically, a bead (or in the case of 10x Genomics' version, a hydrogel) covered in polyT capture sequences is combined in an oil droplet with a single cell. The oil droplet contains reagents that break open the cell and the polyT sequence hybridizes with the polyA sequence on the RNA transcripts. These sequences can be linked back to their original cells and grouped together because the capture sequence includes a cell barcode and a unique molecular identifier (UMI) sequence.
Spatial: The newest kid on the block which uses tissue samples as the input but instead of homogenizing these into single cells, they're treated more like a pathology slide. There are a couple of approaches here with companies using capture array technology, in-situ sequencing, multiplex FISH, or UV microdissection of selected regions of a tissue slide for capture and/or sequencing. They have the added benefit of being able to spatially profile protein locations using fluorescent antibodies; so not only can you use these systems to visualize where the RNAs are expressed in tissues but also the degree to which they create proteins. Which is super cool but also ends up producing some really beautiful images!
Watson and Crick solved the structure of DNA and everyone lived happily ever after, right? Wrong. That was just the opening argument.
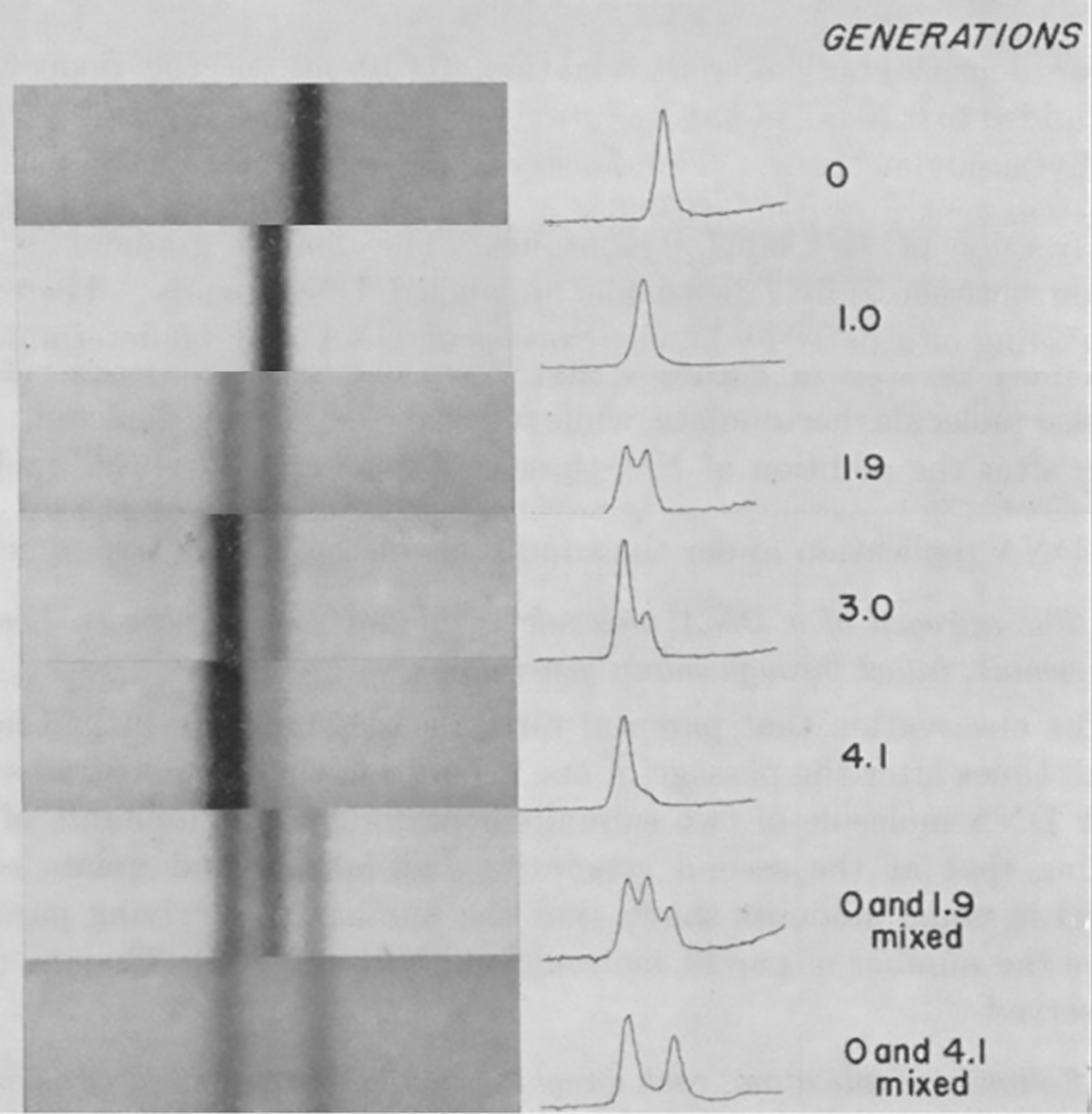
Because once the structure was proposed, everyone needed something new to fight about.
And one of those new topics was how a double stranded DNA helix was copied or 'replicated' to then be passed on to the next generation.
There were three theories for how this might work:
Conservative - A completely new copy of DNA is made that contains no pieces from the parental molecules.
Dispersive - Pieces of the parental molecule are copied and interspersed with fragments of the daughter molecules so the replicated copy is a mishmash of old and new.
Semi-conservative - One strand of a parent molecule serves as a template for creating the daughter strand so one old strand and one new strand end up in the new helix.
Watson and Crick thought the semi-conservative model was the simplest and made the most sense.
But Max Delbrück thought that was impossible because it would require the helix to totally unwind.
He believed that random copying of parent fragments was more likely so he was the champion of the dispersive model.
While the heavyweights were arguing about their theories, Matthew Meselson and Franklin Stahl, two recently minted PhDs, decided to do some actual work.
So, in 1954, they designed a series of experiments to use density gradient ultracentrifugation to settle this latest quarrel.
Now, the method they used might sound complicated but really all they did was extract DNA and spin it really really hard for a very long time - like, 45,000 rpm hard and for 20 hours.
But centrifuging the DNA was only half of the solution, they needed to figure out a way to make ‘old’ DNA strands heavy and ‘new’ ones light.
After a bit of trial and error, they settled on growing bacteria in the presence of a heavy isotope of Nitrogen, N15 and then switching the growth media to regular N14 to see what happened.
If DNA replication was semi-conservative, and not dispersive (random), they hypothesized they would see very sharp bands after centrifugation as the lighter new strands mixed with the old heavy ones.
If there was no banding, and more of a smear, they’d know replication was dispersive.
The results of this experiment are displayed in the figure above and are exactly what would be expected if DNA replicated semi-conservatively.
In Gen 0, everything is heavy, in Gen 1, the helix has one heavy and one light strand, in Gen 2, 50% of the helices are light and 50% are a mix, and by Gen 4, the majority of the DNA helices are composed of light strands.
This simple yet elegant experiment was all it took to convince Max Delbrück that he was wrong and it proved that DNA replication was semi-conservative.
###
Meselson M, Stahl FW. 1958. The replication of DNA in Escherichia coli. PNAS. DOI: 10.1073/pnas.44.7.671
Were you forwarded this newsletter?
LOVE IT.
If you liked what you read, consider signing up for your own subscription here: